Keywords: VPH Solid-phase holographic grating, Transmittance spectrophotometer, Reflectance spectrometer, Czerny-Turner Optical path.
1.Overview
The fiber optic spectrometer can be classified as reflection and transmission, according to type the diffraction grating. A diffraction grating is basically an optical element, featuring a large numbers of equally spaced patterns either on the surface or internally. It is a critical component fiber optic spectrometer. When the light interacts with these grating, disperse into distinct angles determined by different wavelengths through a phenomenon known as light diffraction.
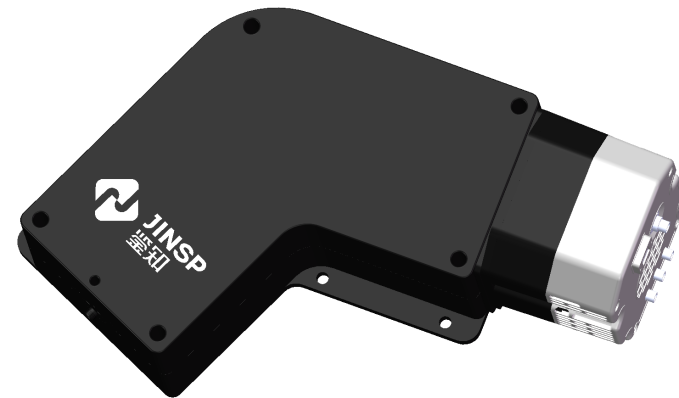
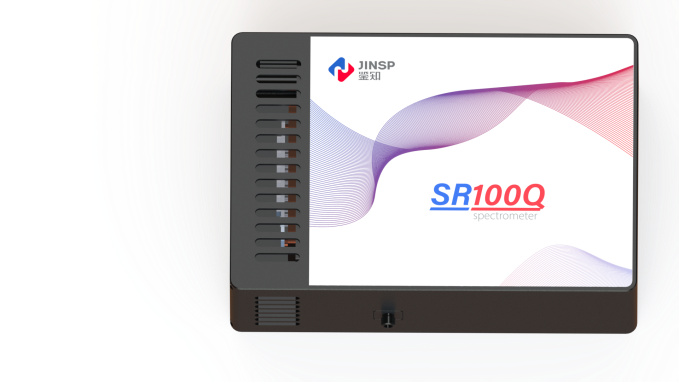
Above: Discrimination reflectance spectrometer (left) and transmittance spectrometer (right)
Diffraction gratings are generally classified into two types: reflection and Transmission gratings. Reflection gratings can be further divided into plane reflection gratings and concave gratings, while transmission gratings can be subdivided into groove-type transmission gratings and volume phase holographic (VPH) transmission gratings. This article mainly introduces the plane blaze grating-type reflectance spectrometer and the VPH grating-type transmittance spectrometer.
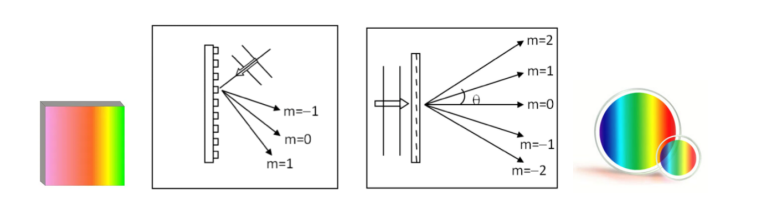
Above: Reflection grating (left) and Transmission grating (right).
Why do most spectrometers now choose grating dispersion instead of prism? It is primarily determined by the grating's spectral principles. The number of lines per millimeter on the grating (line density, unit: lines/mm) determines the grating's spectral capabilities. A higher grating line density results in greater dispersion of light of different wavelengths after passing through the grating, leading to higher optical resolution. Generally, available and grating groove densities include 75, 150, 300, 600, 900, 1200, 1800, 2400, 3600, etc., meeting the requirements for various spectral ranges and resolutions. While, prism spectroscopy is limited by the dispersion of glass materials, where the dispersive property of glass determines the spectroscopic capability of the prism. Since dispersive properties of glass materials are limited, it is challenging to flexibly meet the requirements of various spectral applications. Therefore, it is rarely used in commercial miniature fiber optic spectrometers.
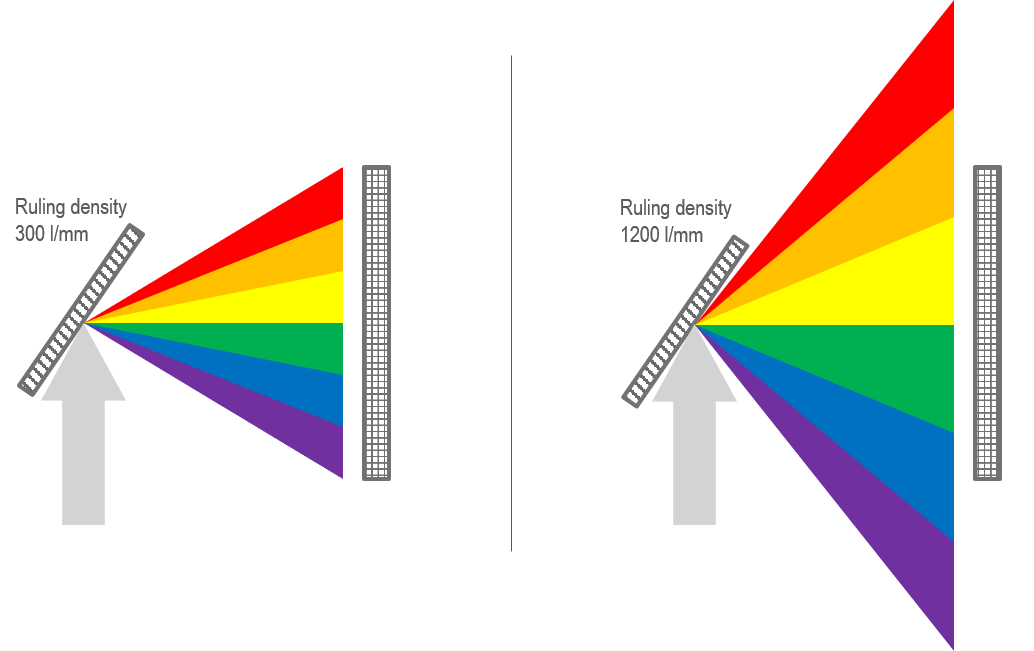
Caption: Spectral effects of different grating groove densities in the above diagram.
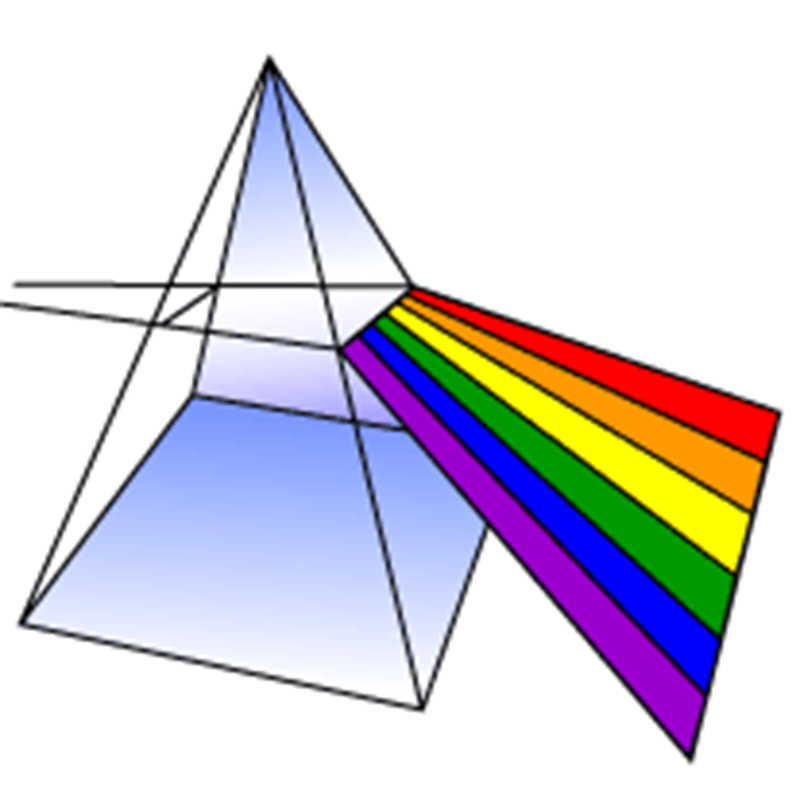
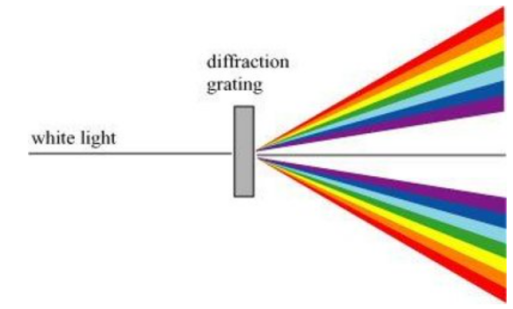
The figure shows dispersion spectrometry of white light through glass and diffraction spectrometry through a grating.
The development history of gratings, startis with the classic "Young's double-slit experiment": In 1801, the British physicist Thomas Young discovered the interference of light using a double-slit experiment. Monochromatic light passing through double slits exhibited alternating bright and dark fringes. The double-slit experiment first validated that light exhibits characteristics similar to water waves (the wave nature of light), causing a sensation in the physics community. Subsequently, several physicists conducted multiple-slit interference experiments and observed the diffraction phenomenon of light through gratings. Later, French physicist Fresnel developed the basic theory of grating diffraction by combining the mathematical techniques put forth by German scientist Huygens, drawing on these results.
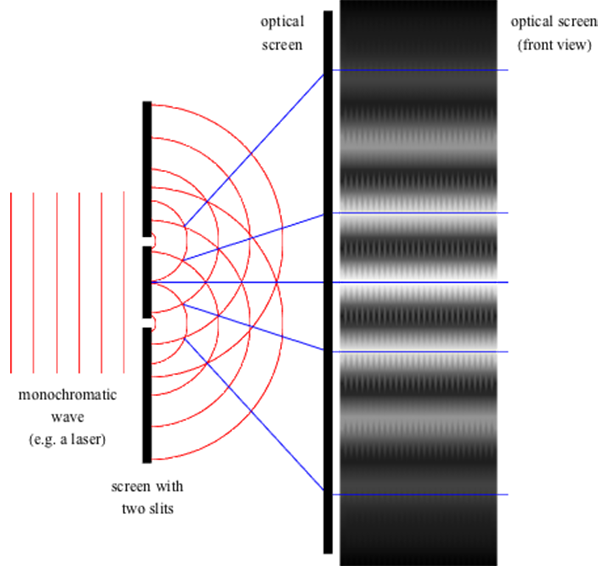
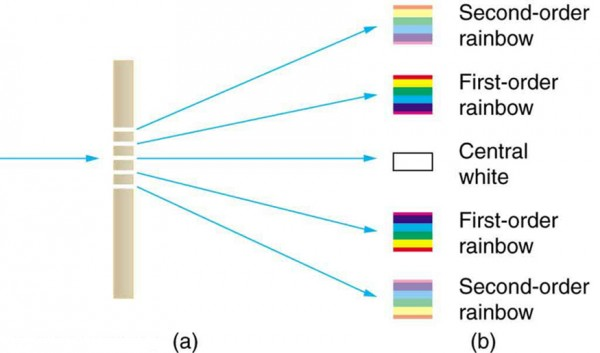
The figure shows Young's double-slit interference on the left, with alternating bright and dark fringes. Multi-slit diffraction (right), distribution of colored bands at different orders.
2.Reflective Spectrometer
The reflection spectrometers typically employ an optical path composed of a plane diffraction grating and concave mirrors, referred to as the Czerny-Turner optical path. It generally consists of a slit, a plane blaze grating, two concave mirrors, and a detector. This configuration is characterized by high resolution, low stray light, and high optical throughput. After the light signal enters through a narrow slit, it is first collimated into a parallel beam by a concave reflector, which then strikes a planar diffractive grating where the constituent wavelengths are diffracted at distinct angles. Finally, a concave reflector focuses the diffracted light onto a photodetector and the signals of different wavelengths are recorded by pixels at different positions on the photodiode chip, ultimately generating a spectrum. Typically, a reflection spectrometer also includes some second-order diffraction-suppressing filters and column lenses to improve the quality of the output spectra.
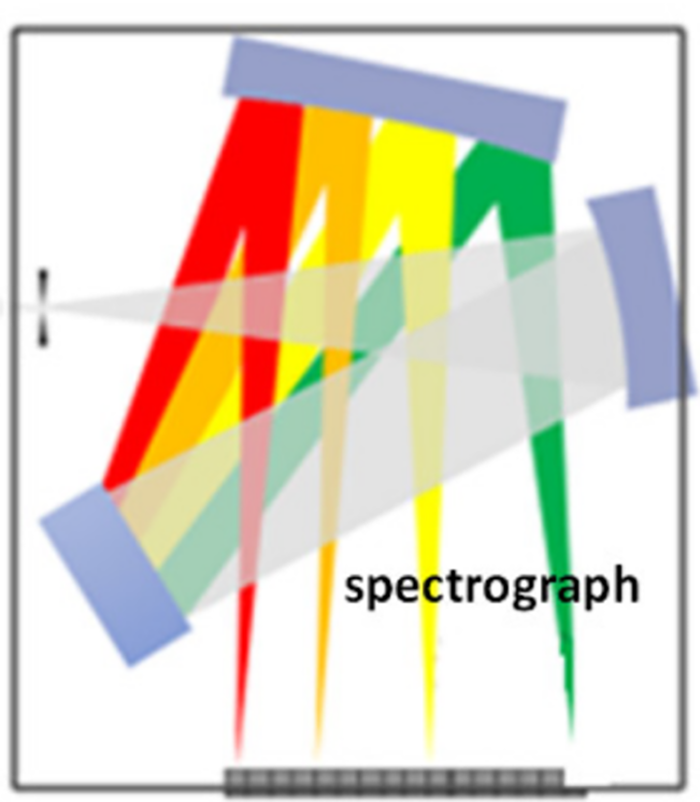
The figure shows a cross-type C-T optical path grating spectrometer.
It should be mentioned that Czerny and Turner are not the inventors of this optical system but are commemorated for their outstanding contributions to the field of optics—Austrian astronomer Adalbert Czerny and German scientist Rudolf W. Turner.
The Czerny-Turner optical path can generally be classified into two types: crossed and unfolded (M-type). The crossed optical path/M-type optical path is a more compact. Here, the left-right symmetrical distribution of two concave mirrors relative to the plane grating, exhibits mutual compensation of off-axis aberrations, resulting in higher optical resolution. The SpectraCheck® SR75C fiber optic spectrometer employs an M-type optical path, achieves high optical resolution up to 0.15nm in the ultraviolet range of 180-340 nm.
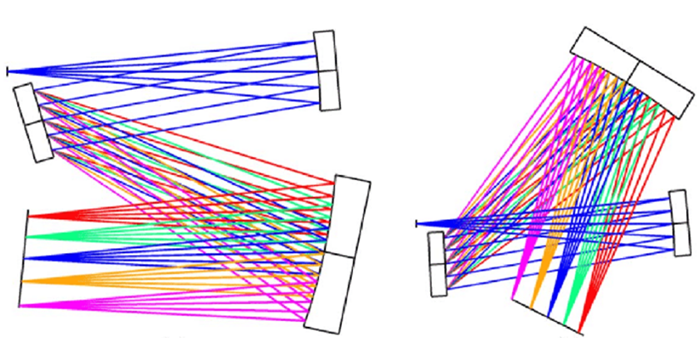
Above: Cross-type optical path/expanded-type (M-type) optical path.
In addition, apart from flat blaze gratings, there is also a concave blaze grating. The concave blaze grating can be understood as a combination of a concave mirror and a grating. Therefore, a concave blaze grating spectrometer consists only of a slit, a concave blaze grating, and a detector, resulting in high stability. However, the concave blaze grating set the requirement on both the direction and distance of incident-diffracted light, limiting the available options.
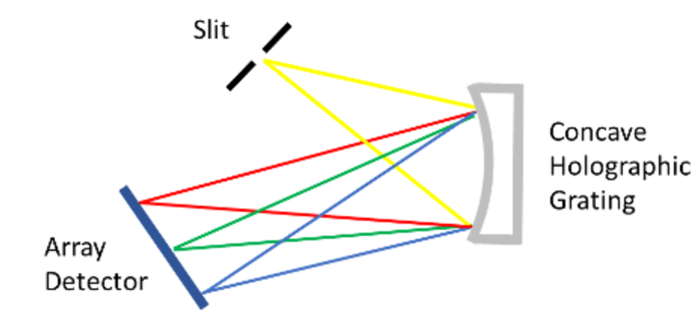
Above: Concave grating spectrometer.
Post time: Dec-26-2023